
My conceptual design will be without the potruding nose but an upward deck where the planes can be launched in a 30 degree angle at the bow. The wings are fixed and a hybrid Blended Wing Body (BWB). The BWB is a type of tailless flying wing design in which the wing and fuselage are blended
together into one seamless body in order to achieve significant improvements in performance
over the conventional aircraft, example shown in Fig. 67. Unlike the flying wing design, in
which the entire body of the aircraft is a wing, the BWB has a fuselage that is designed as a
wing. Therefore, the BWB has a fuselage section that is thicker than the flying wing which
allows it to accommodate more payloads. And, unlike a conventional aircraft, the BWB's
fuselage acts as a lifting body allowing it to generate lift, rather than acting as an interference
component
It will have two twin vertcal stabilizer situated halfway on the wings slanted outward from bow to aft, as the wings will be the whole length of the ship. All engines will be on the other side of the vertcal stabilizer allowing for a clean flight deck and safety for deck personnel.

Turboelectric Distributed Propulsion Engine Cycle Analysis for Hybrid-Wing-Body Aircraft



Visionary Concept: Future Seaplane Transport
5.1. Introduction
During the years of 1950 – 1980, the world was experiencing an exponential growth in
technological advances due to the superpower rivalry between the United States and the Soviet
Union. The aeronautical industry as well got caught in this expansion of technological
exploration. The empirical guidelines during those days were: higher, further, and faster. In the
late 1990’s, this exponential growth reached it maximum peak. The world was experiencing
economical problems, and certainly the aeronautical industry felt its effects. This “out of the
box” thinking that emerged during the postwar era is restricted now to the same problems,
money and social acceptance. Now, according to the European Vision 2020 guidelines, these
have become: more affordable, safer, cleaner and quieter [19]. The old empirical guidelines are
now forgotten, restricting the researcher’s mind to explore radical aeronautical designs.
In order to expand the designer’s mind, a modern vision with more radical, environmentally
efficient, and innovative technologies was created. The new vision is called Future Air Transport
Concept Technologies for 2050 in which the new guidelines will be: safer, quieter, cleaner and
efficient. An efficient concept adapts the early guidelines (higher, further, and faster), with no
restrictions in material, capital or infrastructure for planning, designing, testing, and constructing.
The research addressed aspects such as new ideas in airborne vehicles, including design, new
airport concepts as well as Air Traffic Management (ATM), alternative methods of air transport
system operation and their integration with other transport modes. Let us recall this is just a
radical way of thinking in order to expand the researcher’s mind with no restrictions what so
ever.
The purpose of this chapter was to create an example of this 2050 visionary aircraft concept,
in this case an advance amphibian aircraft. This amphibian aircraft was created on the basis of
the proposed past research, however improving the design into creating a futuristic idea. The new
proposed amphibian adapts advances capabilities such as Unmanned Aerial Vehicle (UAV)
mode, High Altitude and Long Endurance (HALE) capability, water and rescue missions, and
water bomber operations. The advance amphibian aircraft was created from a Blended Wing
Body (BWB) aircraft and converted into an amphibian by adapting the trimaran design concept
and the retracting float system creating an Advance Amphibian Blended Wing Body Aircraft
(AABWBA).
5.2. Review of Literature
The BWB is a type of tailless flying wing design in which the wing and fuselage are blended
together into one seamless body in order to achieve significant improvements in performance
over the conventional aircraft, example shown in Fig. 67. Unlike the flying wing design, in
which the entire body of the aircraft is a wing, the BWB has a fuselage that is designed as a
wing. Therefore, the BWB has a fuselage section that is thicker than the flying wing which
allows it to accommodate more payloads. And, unlike a conventional aircraft, the BWB's
fuselage acts as a lifting body allowing it to generate lift, rather than acting as an interference
component [72].
In 1988, the McDonnell Douglas Company (now Boeing), along with NASA, conducted a
large study on the feasibility of a BWB passenger transport aircraft as an alternative to the
conventional cylindrical tube and wing transport. An initial study performed by Callaghan and
Liebeck in 1990 [73] showed that an 800 passenger BWB, when compared to a conventional
64
transport, cruising at Mach 0.85 with a 7,000 nmi range offered an increase in lift to drag ratio
(L/D) of 40%, and a 25% reduction in fuel burn. Another study performed [74] showed that the
same 800 passenger BWB offered a decrease of 16% in takeoff gross weight (GW) and a
reduction of 35% in fuel required. These improvements in performance are made possible
because the BWB has an extremely low interference drag factor due to the absence of an
interfering fuselage and tail. This allows for the improvement in L/D. In addition, due to the
absence of these components, the BWB is able to achieve a significant decrease in wetted surface
area, which also reduces friction drag.
Another recent and important study on the BWB concept is the European Union sponsored
MOB (Multi-Disciplinary Design and Optimization for Blended Wing Body configurations)
project. The MOB project is a joint project with participation from three aerospace companies,
four research institutions and eight universities throughout the European Union. Publications put
out by the project showed a 10%-19% savings in operating costs for a BWB when compared to
the operating costs of the Boeing 747-400 aircraft [75].
Fig. 67: Example Blended Wing Body Aircraft [72]
5.3. Design Selection
5.3.1. Input Parameters
It was analyzed as the best choice in terms of productivity to use an aspect ratio of AR = 14
and W/S of 195 kg/m2
on the BWB aircraft. The higher AR and relatively low W/S equates to a
lower GW through requiring a lower engine thrust and lower fuel usage.
AR was varied between values of 8 to 14 and W/S was varied between 90 kg/m2
and 400
kg/m2
. The values of AR were chosen because research showed that these were typical values for
flying wings [76]. The values of W/S were chosen because this range of W/S was typical for
HALE type aircrafts.
An important point to notice is that as AR increases, GW decreases. This is in part due to the
decrease in wing area that higher aspect ratios have. Another important point to notice from this
is that the lowest GW occurs at W/S of 195 kg/m2
. Since it is always important to optimize and
lower the weight of a new aircraft, a W/S of 195 kg/m2 was selected. Using this information, an
AR of 14 would result in the lowest amount of thrust required. This combination of lowest GW
and lowest thrust required with an AR of 14 and W/S of 195 kg/m2 became the selected
configuration for the BWB.
65
The unfortunate side effects of having such a large AR are structural effects due to bending,
manoeuvrability, parasitic drag and low internal volume for fuel. Longer wings simply have
more bending stress and lower roll rates, however these two drawbacks were deemed to be worth
the risk due to advanced material selection which offers better structural strength and lower
structural weight. The lower roll rates would also not affect the designed mission very much as
the mission is not one that requires high maneuverability.
An increase in parasitic drag is partially offset by a reduction in induced drag from having a
longer wing span, and especially offset by the huge reduction in equivalent at plate area due to
the BWB configuration. Values calculated for the amount of fuel required for the designed
mission indicate that the design has plenty of room for fuel within the wing of the plane.
5.3.2. Fuselage Thickness
In order to fit sizable payload into the fuselage, a wing thickness ratio of 17% was used. This
value is much higher than typical transonic airfoils and it is the upper limit of thin airfoil theory
[76].
5.3.3. Airfoil
The BWB design is a tailless aircraft, and therefore a reflexive airfoil is required to produce a
positive pitching moment to counteract the negative pitching moment of the wing. The NACA
6400 series airfoils was selected due to the ready availability of data (drag polar, moment arm,
etc) and because the cambered airfoil can achieve a higher lift coefficient at lower angles of
attacks [27]. While the NACA 6400 series is not a reflexive airfoil, the elevons can be trimmed
in such a way to have a negative deflection, therefore creating a pseudo reflexive airfoil without
sacrificing interior cabin space.
For the fuselage section of the aircraft, a NACA 6417 and 6416 were selected to utilize the
maximum 17% t/c available. The NACA 6417 was placed in the middle of the aircraft, with the
NACA 6416 on the outer edges of the fuselage. A NACA 6410 was then chosen to be the airfoil
shape at the wing tips. The entire aircraft was then lofted together in such a way that the entire
aircraft is one blended body.
The NACA 6410 airfoil has a CLmax of 1.3. From all of the calculations, this value was
sufficient given the available thrust. Therefore, high lift devices such as slats were not used. In
addition, high lift devices such as flaps could not be used because it increases the negative
pitching moments of the aircraft [76] which cannot be counteract because it does not have a tail.
5.3.4. Wing Sweep (Λ)
Boeing's 800 passenger BWB used a Λ of 36o
[76] from the quarter chord, which swept the
tips of the wings back behind the engines, providing the inherently unstable BWB with increased
stability and control. One of the drawbacks to having a highly swept wing is that air flow over
the wing begins to divert and flow over the wing toward the tips in a diagonal manner, instead of
in a straight line from front to rear. In order to address this problem, wing fences were
constructed on the wing in an effort to redirect flow back into a better wing front to rear manner.
Fig. 68 shows a top-down view of the BWB, showing the wing and wing fence design.
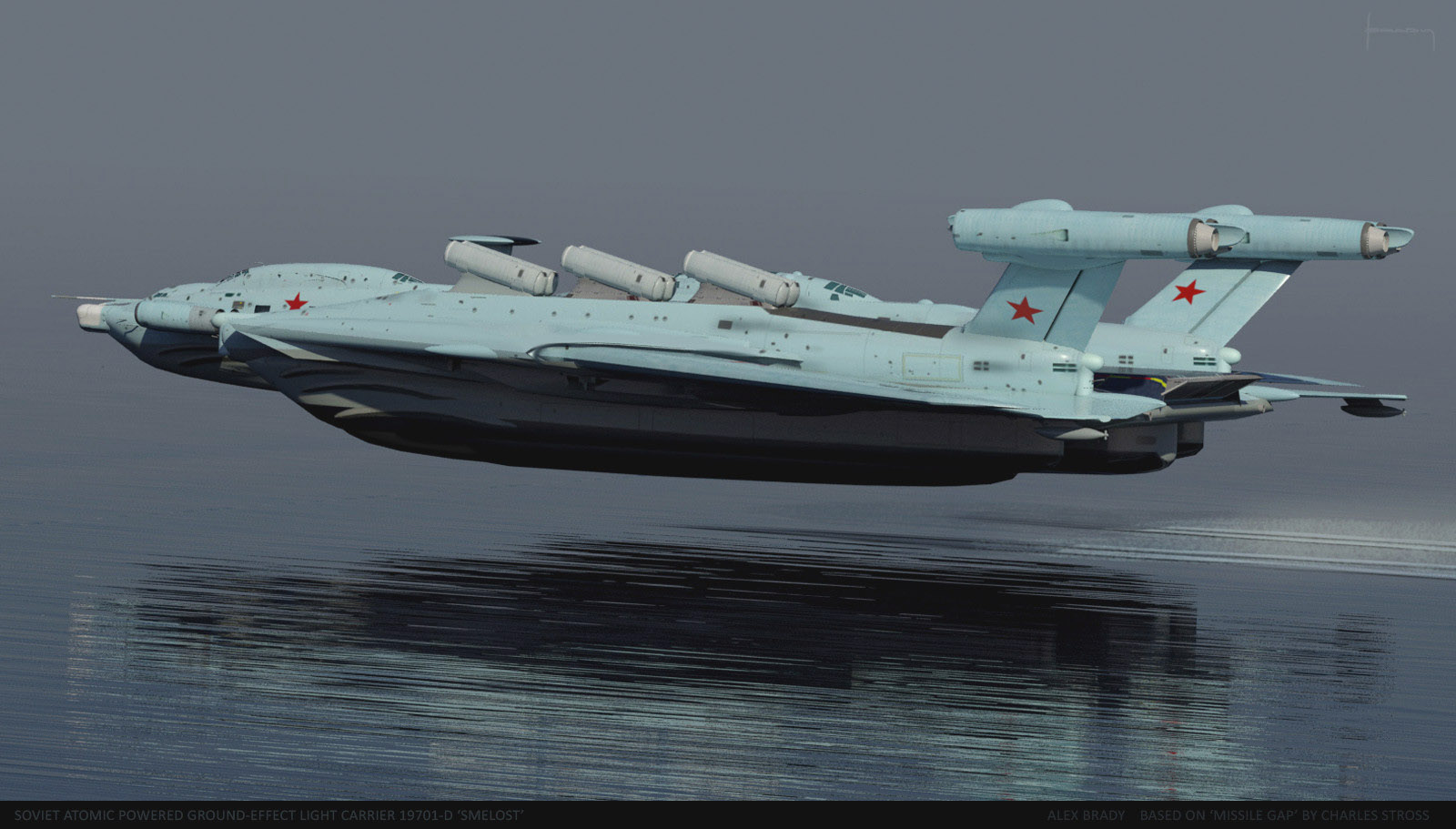

Keywords: soviet fold wing ocean landing floating flying aircraft carrier with landing pad illustration render by concept artist alface killah artstation.com portfolio image samples boac
No comments:
Post a Comment